Fall 2015
Research Rotation Writeup
by Meg Smith (ESS graduate, 2015)
At the end of March, 2015, I hopped in my car and drove 14 hours from Seattle, Washington to Mountain View, California. After what felt like days on the road (nobody ever said Toyota Corollas were comfortable), I arrived in Mountain View a couple hours after sunset on my second day. I was excited to start my research rotation.
Completing a research rotation is a requirement for receiving both the graduate certificate in Astrobiology and the dual PhD in Astrobiology. To successfully complete a research rotation, a student must work on an astrobiology-related project outside his/her main field of research for one academic quarter.
For my graduate research, I have used computer models to analyze the atmospheric chemistry of Mars and the early Earth (my adviser is David Catling, in the department of Earth & Space Sciences), so for my research rotation, I wanted to get off my computer and get into a lab. Through the Virtual Planetary Laboratory (run by UWAB’s Professor Vikki Meadows), I had been introduced to Dr. Niki Parenteau during the fall of 2014. Niki is a researcher at the SETI Institute and NASA Ames Research Center in Mountain View, CA. After we had a discussion at the American Geophysical Union (AGU) conference in winter of 2014, Niki graciously agreed to supervise me for my rotation at NASA Ames. While our research methods are very different (theoretical modeling for me and lab- and field-based experiments for her), we came together for a rotation because of common interests in how microbial life interacts with the atmosphere.
Microbial life modulates the chemistry of an atmosphere by producing gases that can potentially persist for long periods of time. These gases are called biosignature gases, or “biosignatures” for short. The majority of recent research on biosignatures has focused on O2, and its photochemical byproduct, O3. There is a problem; however, with only considering these two gases. Oxygen is abundant in today’s atmosphere, but during the first half of Earth history, O2 and O3 only existed in trace amounts (see Figure 1). Instead, more reduced gases, like CO2, CH4, and H2 dominated the atmosphere. To characterize biosignatures from the reduced era of Earth’s history, one needs to look to modern anaerobic organisms – organisms that exist and thrive in the absence of O2. Anoxygenic photosynthetic bacteria are one class of anaerobes—they are organisms that conduct photosynthesis, but instead of splitting water and producing O2 as a byproduct like oxygenic photosynthetic bacteria, they oxidize reduced gases like H2 or H2S. Light and reduced dissolved species were definitely present on the early Earth, and these organisms were as well (the first evidence for anoxygenic photosynthesis is over 3 billion years old). Niki specializes in characterizing these organisms. Our goal, for my rotation, was to use a laboratory instrument to identify gases produced by anoxygenic photosynthetic bacteria.
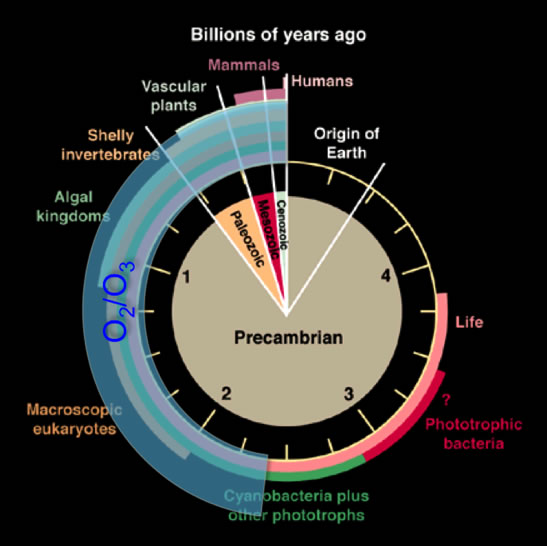
Figure 1: History of life on Earth, shown as a clock. O2 and O3 were virtually absent in Earth’s atmosphere during the first half of its history. The image is modified from Des Marais (2000).
Prior to my arrival at NASA Ames, the lab in which Niki works had recently acquired an instrument that detects dissolved gases. This instrument, a mass spectrometer, has a probe that is inserted into a liquid media. When the instrument is turned on, it ingests dissolved gases, ionizes those gases, and uses a detector to measure the amount of each gas with a distinct mass. For the bulk of my research rotation, I learned how to use the instrument, calibrated the instrument, and measured dissolved gases in an environmental sample and a pure culture; both of which contained anoxygenic photosynthetic bacteria.
The environmental sample contained a mixture of organisms, including purple sulfur bacteria (anoxygenic photosynthetic bacteria that can use H2S as an electron donor and look purple in color). On one of the few days I was not in the lab, Niki and I collected this sample at a hot spring site in northern California called Wilbur hot springs (see Figure 2). Being in the field at Wilbur was a blast for me because I learned multiple techniques for collecting samples, and I was able to be outside in the sunshine all day (when you live in the Pacific Northwest, you learn to love sunny days). It was fascinating to see the diverse microbial communities thriving in scalding, toxic water.
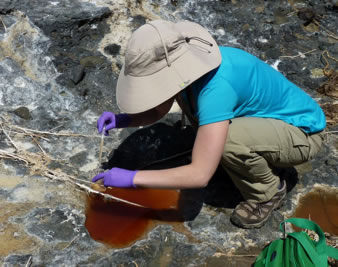
Figure 2: Meg collecting a sample containing purple sulfur bacteria from Wilbur Hot Springs in northern California.
The pure culture consisted of a purple nonsulfur bacteria that was isolated by Niki from a sample she had previously collected at Lassen Volcanic National Park. The culture was already in place when I arrived at Ames, but that did not stop me from being able to work with it. Among other things, I created media for the culture and transferred the culture to the new media. Microbiologists need to transfer cultures periodically because microbes will end up contaminating their containers with waste products, which could then kill the microbes.
In order to characterize gas production, I conducted two experiments for each of the samples. First, I used the mass spectrometer to measure the dissolved gases in the samples under the normal lab conditions. I discovered both the environmental sample and the pure sample contained a lot of water (mass 18), nitrogen (mass 28), and carbon dioxide (mass 44). There were also two unknown gas detections at mass 32 and mass 19. The detection of mass 32 may have come from a sulfur atom that had been broken off of dissolved H2S. The mass 19 species remains unidentified. Second, I conducted an experiment for each sample in which I exposed the sample to oscillating light and dark conditions (see Figure 3). Light was provided using a 200 W Tungsten bulb, and darkness was simulated by covering the sample with a dark cloth. I observed distinct changes in gas speciation as the light was turned on and off. The most interesting – and unexpected—change was an increase in CO2 abundance when the light was turned on and a decrease when the light was turned off. This was odd because I expected phototrophs to consume CO2 when actively metabolizing (in light), which would cause the CO2 abundance to decrease. Regardless of the cause of this CO2 change (Niki thinks CO2 may have been produced by photoautotrophs functioning as photoheterotrophs), it is interesting because CO2 could be a biosignature that nobody has considered before. With that said, CO2 is also produced by abiotic processes, so it is not clear if biologic CO2 production would have been important on the early Earth. This brings up an important point -- the experiments I completed with Niki are a critical, but early, step in characterizing biosignatures from anoxygenic photosynthetic bacteria.
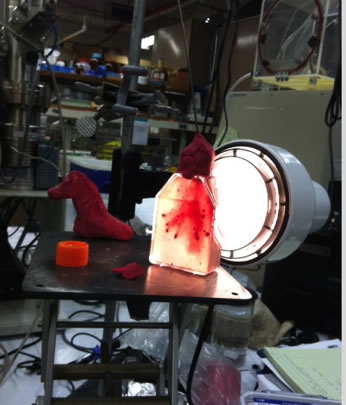
Figure 3: Exposing the purple non-sulfur bacteria to light. The sample is on the right, and our lab mascot, a unicorn head made of modeling clay, is on the left.
After almost quarter’s worth of work in the lab and in the field, before I realized it, I was in the last week of my rotation. On the last day, I packed up my car once again and got ready for the ride north. As I hit the road to repeat the 14 hour drive back to Seattle, I thought to myself that I had only one regret—that I cannot do another rotation!
Reference:
Des Marais, D. J., 2000. Evolution - When did photosynthesis emerge on earth? Science. 289, 1703-1705.
Return to Front Page |