Things can change a lot in sixty years! I grew up with a fascination both with invertebrates and water, but living in northeastern Ohio, the invertebrates I knew were insects and the water was the fresh stuff in Lake Erie. My amateur interests in collecting insects turned to a fascination with their biology while I was an undergraduate at Notre Dame, and solidified into a life-long career with my PhD from Harvard. I thought that insect diversity was rich enough to keep me occupied for a lifetime, yet sixty years later I find myself studying their relatives, the crustaceans.
In the last sixty years there has also been a change in our understanding of the relationship of the various classes within the Arthropoda, the phylum of animals with an exoskeleton and jointed limbs. Early on, I had learned that there were three main branches in the arthropod family tree – the Chelicerata (spiders and their kin), the Crustacea, and the Tracheata (arthropods with single-branched limbs including insects, centipedes, and millipedes). Over the decades, though, a number of lines of evidence including the increasing power of molecular tools upended this view and showed that the insects were not related to the centipedes and millipedes after all, but arose from within the Crustacea. The combined group is now called the Pancrustacea. This revision of arthropod relationships is similar to our new appreciation of relationships within the vertebrates, as we now know that birds are actually a group of flying dinosaurs! As it turned out, I had actually been working on a specialized type of crustacean through my entire career and did not know it! Our research on crustaceans began in earnest in the Spring of 2003 when Lynn Riddiford and I accepted an invitation from Dennis Willows to teach a Research Apprenticeship at FHL. By the end of the quarter, we had bought a house on the island, and were “hooked.”
Much of my insect research has dealt with what happens to the nervous system during the dramatic upheaval of metamorphosis. We found that neurons are born, killed or recycled as the nervous system of a crawling caterpillar is rebuilt into one appropriate for a flying moth. We showed that these changes were controlled by steroid hormones and that the insect nervous system provided a relatively simple model for understanding cellular aspects of how steroid hormones control brain development and function. Though intellectually satisfying, these pursuits moved me away from what fascinated me about Biology in the first place: the diversity of organisms and the myriad ways that they evolved to meet their basic biological needs.
Things changed in 2007. An offer from the Howard Hughes Medical Institute to become a Group Leader at their new Janelia Research Campus in northern Virginia provided the opportunity to try to develop a synthetic view of nervous systems and the rules that govern their development, function and evolution. Both crustaceans and insects evolved a rich diversity of body forms as they became dominant groups in aquatic, terrestrial and aerial environments. They share a segmented body plan made up of repeating units, with each segment bearing a pair of appendages and a ganglion containing the neurons to control these appendages. The segments along the body show regional specializations with the head appendages involved in food manipulation while those on the next body segments control locomotion. In the insects, for example, the latter are the thoracic segments that bear the walking legs and wings. My goal at Janelia was to determine how the nervous system was able to cope with the diverse types of legs and wings that had emerged during the course of insect evolution.
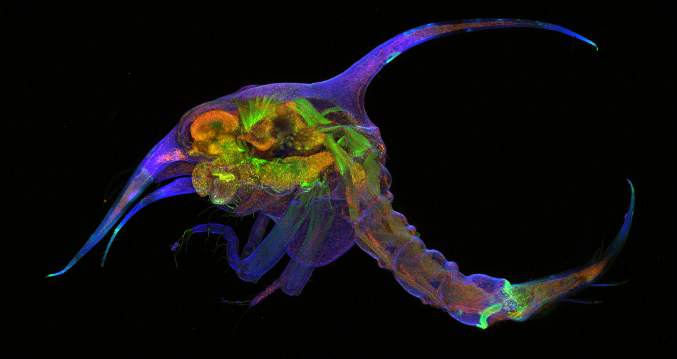
Our previous studies on insects showed that the evolution of flight was accompanied by a 3-fold increase in the number of neurons in each thoracic segment, presumably to deal with the complexities of flight. Even for a relatively simple insect ganglion, the neurons number in the thousands, making analysis and comparisons a numerical nightmare. It turned out, though, that the animal made it easier for us because these thousands of neurons are produced by a stereotyped set of 30 pairs of neuronal stem cells called neuroblasts. Each neuroblast produces a fixed set of neurons which we call its lineage. Using the genetic tools available for the fruit fly
Drosophila melanogaster, we determined the properties and functions of the neuronal lineages produced by each of the 30 types of neuroblasts. We found that each lineage formed a functional module that fit together with the modules produced by other neuroblasts to make the computational networks used for walking and for flight. Since other workers had shown that the same set of segmental neuroblasts was found throughout the insects and also in the crustaceans, we hoped that our Drosophila data would provide the Rosetta Stone (master translator) for understanding how nervous systems were organized and functioned throughout the Pancrustacea.
Fig. 1 (above): Confocal image of a zoea (crab) larva showing the exoskeleton (blue), muscle (green) and cell nuclei (red).
Our comparative work on insects shows us how these neural modules are modified to accommodate various modes of flight, but our return in 2016 to FHL and its diversity of marine life enabled us to expand into crustaceans and the question of how these modules might relate to the evolution of legs and of walking. We have been using confocal microscopy to study their skeletons and musculature (Figure 1) and as well as their nervous systems. We have focused on the most diverse and successful group of crustaceans, the Malacostraca, that includes shrimp, crabs and lobsters. Some have simple limbs like the ‘paddles’ of
Nebalia, but most have branched and segmented legs with muscle groups in each segment (Figure 2). These limbs differ in their function in the various crustacean groups. The inner branch of the branched limb is destined to become a walking leg although in the mysids and the euphausiids, they are used for prey capture rather than for walking. They serve as walking legs in amphipods and in shrimp, such as
Crangon, but it is only the latter that walks with highly refined and coordinated leg movements. The outer branch of the limb is adapted for swimming. It is well developed in mysids, reduced in the euphausiids, and lost in the amphipods and in
Crangon. The reduction and loss of the swimming branch is accompanied by the abdominal segments taking over swimming function for the animal.