Biomedical Nanomagnetics
A rapidly evolving, multidisciplinary field that impacts engineering, physical science and medicine, Biomedical Nanomagnetics aims to detect disease at the earliest possible time through advances in imaging and diagnostics. It also addresses the challenge of delivering treatment at the right place and at the right time while minimizing unnecessary exposure through targeted therapy with a triggered release. Translational applications in our group include cardiovascular angiography, molecular imaging of cancer, and establishing chemical pathways in neuroscience and renal ischemia.
MPI is a new medical imaging technology, in which Iron Oxide Nanoparticle Tracers and safe magnetic fields are used to generate 3D images in real time
(click here to see a video produced by Philips, MPI’s inventors, which explains basic MPI principles and potential applications.). With excellent sensitivity and spatial resolution, MPI shows great promise for treatment of heart disease and in molecular imaging applications such as early cancer detection. Critically, MPI requires iron-oxide tracers with finely-tuned size to achieve good image quality. We model tracer magnetic properties to determine the ideal size for desired imaging conditions [1] and then synthesize and process optimized, biocompatible MPI tracers with outstanding MPI performance [2].
Prof. Krishnan, Dr. Ferguson, and Amit Khandhar have recently spun out a company, LodeSpin Labs, to commercialize their MPI tracer technology.
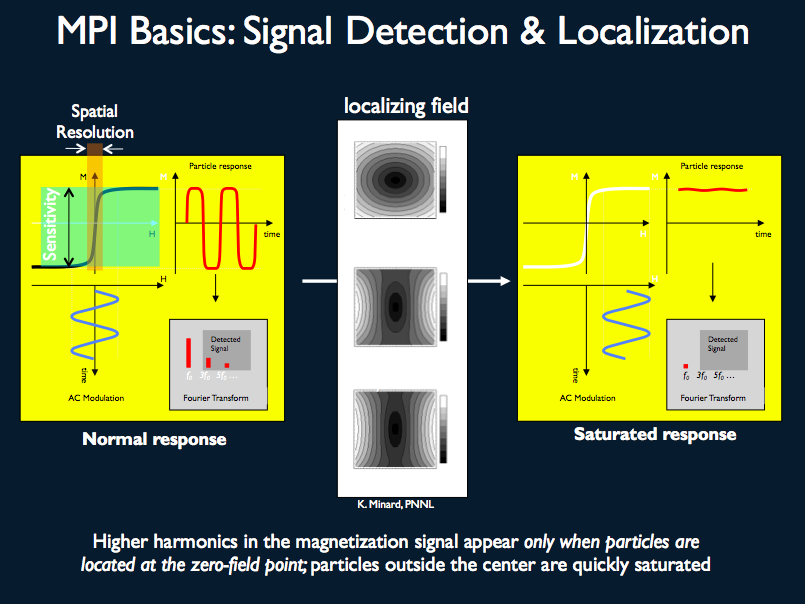
[1] Ferguson, R., Minard, K., & Krishnan, K. (2009). Optimization of nanoparticle core size for magnetic particle imaging.
Journal Of Magnetism And Magnetic Materials,
321, 1548–1551. doi:10.1016/j.jmmm.2009.02.083
[2] Ferguson, R. M., Khandhar, A. P., & Krishnan, K. M. (2012). Tracer design for magnetic particle imaging (invited).
Journal of Applied Physics,
111(7), 07B318. doi:10.1063/1.3676053
[3]Ferguson, R. M., Minard, K. R., and Khandhar, A. P., & Krishnan, K. M. (2011). Optimizing magnetite nanoparticles for mass sensitivity in magnetic
particle imaging.
Medical Physics,
38(3), 1619–1626.
Engineered MRI Contrast Agents for
In vivo Sensing of Biological Uptake
Contrast agents play an important role in improving the diagnostic capability of Magnetic Resonance Imaging (MRI). In general, paramagnetic ions such as Mn or Gd are used to provide contrast in T1-weighted images, while superparamagnetic nanoparticles are utilized in T2-weighted imaging. We are developing MnO@SiO core-shell contrast agents that have been tested to engineer a delayed increase in MRI T1 relaxivity caused by cellular uptake via endocytosis into acidic compartments. Several known surface modifications enable us to link our nanoparticles with specific molecules to form molecular probes that can be used as in vivo diagnostic tools. The delayed enhancement may have benefits for targeting MRI contrast to specific cells and surface receptors that are known to be recycled by endocytosis. These pH-sensitive contrast agents have been applied to studies of thalamo-cortical connections in neuroscience, renal ischemia and stroke models in animals. This work is in collaboration with Dr. Alan Koretsy and his group at NIH-NINDS.
Tailored Nanoparticles for Magnetic Fluid Hyperthermia
Magnetic fluid hyperthermia (MFH) is the localized heating of cells and tissues using superparamagnetic magnetite (Fe3O4) nanoparticles (MNPs). The goal in MFH is to localize heating therapy (3-5C above physiological temperature) to disease sites such as tumors and atherosclerotic plaques, while minimizing damage to healthy tissues from heating and MNP toxicity. In order to achieve maximum therapeutic outcome with minimal MNP dose, the physical and magnetic properties of governing magnetization reversal must be tailored to the AC-field conditions.
Cytotoxicity, Biodistribution & Clearance
Surface coatings play a critical role in the colloidal stability and biocompatibility of nanoparticles. PEG coatings in particular, are non-fouling, non-immunogenic and increase blood half-life. We test the in vitro cytotoxicity of nanoparticles using complementary cell viability and toxicity assays. Furthermore, biodistribution study in mice show that nanoparticles are taken up in the liver and spleen, and over 7 days, show gradual clearance.
Intracellular Localization, Fate, & Magnetic Dynamics
Superparamagnetic iron oxide nanoparticles are strong candidates for cell tracking applications in Magnetic Particle Imaging (MPI). Quantitative and continuous tracking is only possible with consistent magnetic signal in cellular environments, which is not the case with current nanoparticle and coating formulations. This loss of performance has been determined to orginate from interparticle magnetostatic interactions. Current studies are in progress to alleviate or fully recover the loss of signal through steric control of polymer coatings on nanoparticles.