Walking the Tightrope Between Stability and Change
by Dianna K Padilla
Dianna is a professor in the Department of Ecology and Evolution at Stony Brook University on Long Island in New York. She first came to Friday Harbor Laboratories when she was an undergraduate at the University of Washington. She has been a regular research visitor since that time.
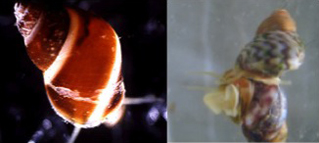
Fig. 1: Lacuna vincta and Lacuna variegata. These snails are typically 3-9 mm long.
For animals and plants living on the shore, the only constant is change. Some of this change is natural and predictable. Each day the tides ebb and flow, covering and exposing organisms. Changes in temperature and sunlight follow seasonal changes. There are also year-to-year changes when normal weather patterns are disrupted. One example is the El Niño Southern Oscillation (ENSO), which brings hot sunny weather to the San Juan Islands. However, some changes are a result of human activity, including the introduction of new species from other areas, or increased greenhouse gas emissions that have caused climate change and are now causing ocean acidification. One of the questions we are trying to address in my research lab is: can and how do organisms respond to changes in the environment while maintaining all of their normal functions? Or, how do organisms walk the tightrope between stability and change?
My students and I have spent a lot of time looking at snails that experience changes in their environments, and examining their ability to respond to that change in short periods of time – a process that we call phenotypic plasticity. The easy way to think of a phenotype is how an organism looks. "Phenotypically plastic" animals can change how they look in response to different environments. We are particularly interested in animals that can and do respond to short-term changes in their food availability or risk of predation.
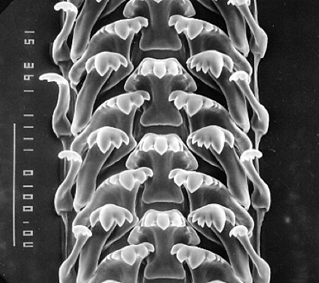
Fig. 2: The radula ribbon with repeated rows of teeth. The scale bar on the left side is 100 microns in length.
Much of my work over the past 25 years has focused on very cute but tiny snails in the genus Lacuna (Figure 1), which live in seagrass beds and on macroalgae such as kelp. When I was working on my PhD research, I studied tooth shape in snails and how it affects their ability to feed on different types of food. Yes, snails do have teeth; in fact, each snail has hundreds of them! Their teeth are attached to a long ribbon (the radula) and are rasped across surfaces when snails feed (Figure 2). As the teeth at the mouth end of the radula wear down with feeding, new teeth are constantly developing at the other end of the radula, producing a never-ending conveyor belt of teeth. I discovered that the shapes of snail teeth have a big impact on how effectively they can eat different types of food. Different tooth shapes would be expected for snails feeding on fleshy algae, like kelp, than those that feed on the film of diatoms and other microalage covering the surface of eelgrass leaves. So, what kinds of teeth should snails that feed in these two environments produce? We would predict that snails feeding on kelp should have pointed, cusped teeth, making it easier for them to tear the tissue of the kelp. However in eelgrass beds, broad, blunt-shaped teeth would be best for removing diatoms from the surface of leaves. When we looked at the snails, that is indeed what we found (they read my papers!). There was only one problem. The shapes of snail teeth are typically used to identify species; they are supposed to be the same for all individuals in the same species. We had two different species of snails in both habitats, and both produced teeth that matched their food. This called for experiments!
We collected snails from the eelgrass bed at the mouth of False Bay on San Juan Island, where snails have blunt-shaped teeth, and from the kelp and macroalgal bed to the south of False Bay (inside the marine reserve, a research site called Mar Vista). At the Friday Harbor Laboratories, we ran a series of experiments on our snails. We gave them different types of food and then examined their teeth. What did we find?
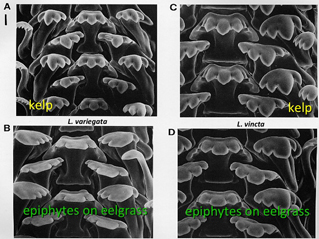
Fig. 3: The results of one of our experiments: snails fed diatoms on eelgrass produced blunt shaped teeth (B and D) while those fed kelp produced pointed teeth (A and C).
Snails from both species that were fed diatom-covered eelgrass, irrespective of their previous diet, produced blunt-shaped teeth. Similarly, snails that were fed kelp, irrespective of their previous diet, produced pointed-shaped teeth (Figure 3). Thus, snails can have phenotypically plastic tooth shape, and can respond to changes in their diet within days of moving to a new environment. These snails can drift in the water on mucus parachutes, moving among habitats. Based on our samples in the field, on a given day up to 20% of snails at a site can be recent transplants from the other habitat type. For these recent transplants, there will be two different shapes of teeth along the radula. The shape of the older teeth (towards the mouth) will reflect the old habitat, while new teeth at the other end of the radula reflect the best tooth shape for the current habitat.
We have also been looking at how snails respond to the presence of their predators. For Lacuna, chemical odors from their predators make them parachute more, probably with the hopes of landing somewhere with fewer predators. Snails that are too big to parachute (and must tough it out if predators are in the neighborhood) change the shape of their shells, making them thicker and harder for predators, especially crabs, to break. This change in shell thickness and shape appears to be a byproduct of behavioral changes in snails. When large snails like the whelk Nucella lamellosa smell chemical cues from their crab predators, they hide in cracks and crevices and reduce the amount of time they spend on the shore feeding. Snails that are given less food produce thick shells that are the same shape as those exposed to predators such as the red rock crab. We will soon be investigating other species of snails, including Lacuna, to see how exposure to higher risk of predation affects their shells.
Relevant References:
Grünbaum D. and D.K. Padilla. 2014. An integrated modeling approach to assessing linkages between environment, organism, and phenotypic plasticity. Int. & Comp. Biol.: 54 (323-335).
Miner B.G., Sultan S.E., Morgan S.G., Padilla D.K., and R.A. Relyea. 2005. Ecological consequences of phenotypic plasticity. Trends in Ecol. & Evol.: 20 (685-692).
Padilla D.K., Daniel T.L., Dickinson P.S., Grünbaum D., Hayashi C., Manahan D.T., Marden J.H., Swalla B.J. and B. Tsukimura. 2014. Addressing grand challenges in organismal biology - the need for synthesis. BioScience: 64 (1178-1187).
Padilla D.K. and B. Tsukimura. 2014. A new organismal systems biology: how animals walk the tightrope between stability and change. Int. & Comp. Biol.: 54 (218-222).
Look for Tide Bites in your inbox at the start of each month! To view previous Tide Bites, visit the FHL archive page located on the FHL website.
