Fantastic Voyage
By Jason Hodin
Scientists and science enthusiasts alike are familiar with many of our iconic sea shore creatures: crabs, worms, sea anemones, sea stars, sea urchins, tidepool fish and seaweeds. What is less appreciated is that almost all of these creatures lead a dual existence, one part of which occurs at a microscopic stage.

In animals this stage is called the larval phase, and while most shoreline animals move very little as adults, their larvae can travel vast distances. In some cases they can even cross oceans!
While this may seem a good strategy for animals and non-animals to colonize new habitats, it also creates a serious problem when suitable habitats are few and far between. Imagine our purple sea urchin, which is adapted to high-energy rocky shores on the outer coast of Washington. Its larvae, released into the open ocean, are first tasked with finding food in the plankton and surviving numerous predators such as jellies. During this time, they grow from a mere ball of embryonic cells through a series of complex larval stages that look nothing like the adult (see image), finally reaching a mysterious stage called competence where they are capable of completing their metamorphosis back to the sea floor. But what if the currents have carried them far offshore in the meantime? In that case they need to somehow return to the shore and, crucially, recognize when they have arrived there. And not just any shore is acceptable for purple urchins: it needs to be rocky, high-energy shoreline.
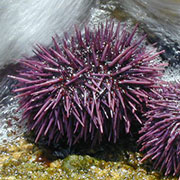
The odds seem to be poor that a given larva will successfully navigate these challenges, and the life cycle patterns of coastal organisms in general are well adapted to these long odds; purple urchins are no exception. A single spawning of a purple urchin female can result in the release of millions of embryos, and the vast majority of the ensuing larvae never survive to reproduce as adults (or we would be completely overrun by urchins)!
The flipside of these sweepstakes-like odds of survival is that any advantage a given larva can muster could greatly improve their chances of survival and reproduction. My colleagues and I therefore predicted that purple urchin larvae would be at a great advantage if they could recognize that they are approaching the shore. And what is one feature of the oceanic environment that would be a sure-fire indicator to a larva that it is approaching the shore? A huge increase in the level of fluid turbulence, characteristic of waves breaking on the shoreline!

So we set out to test this idea using a laboratory apparatus known as a Taylor-Couette Cell, which can generate precise levels of fluid turbulence at the scale of a larva. When we exposed late-stage purple urchin larvae to a mere 60 seconds of intense, surf-zone levels of turbulence, it greatly increased the likelihood that these larvae would make their one-way transition from floating larva to crawling juvenile. Indeed, we discovered that larvae that we previously considered unresponsive to the smells of their juvenile habitat suddenly became responsive after turbulence exposure. In other words, turbulence makes larvae competent to settle down as juveniles!
With funding from the National Science Foundation, I have continued this work at Friday Harbor Labs in collaboration with my colleagues in California to better characterize this response, and to make broader evolutionary comparisons. For example, we have recently found that the pink or fragile sea urchin — a deep sea cousin of the purple sea urchin — is not activated in this way by turbulence. And in collaboration with Professor (and frequent FHL visitor) Tony Pires of Dickinson College, I found that the slipper shell Crepidula fornicata — an invasive mollusk — shows a response similar to purple urchins but at a much lower level of turbulence; this matches its lower-energy, moderately protected intertidal adult habitat.
As with most interesting issues in biology, we are left with far more questions than answers. For example: what cellular mechanisms do larvae use to sense turbulence, and how do they translate that information into settlement behavior? How do larvae integrate their various sensory cues to make good decisions at the crucial settlement transition? And, can we figure out a way to recreate our intriguing laboratory observations in the field? My collaborators and I are developing plans to try to answer these and other questions, and hopefully one day understand how larvae accomplish their amazing and unlikely journeys, year after year, in oceanic habitats worldwide.